311 Irani Oxi Engineering Complex
Department of Mechanical Engineering, Faculty of Engineering and Architecture, American University of Beirut
-
Microfluidic transistors for analog microflows amplification and control
-
Microfluidic mixing using pulsating flows
-
Reduced-Order Modelingof Low Mach Number Unsteady Microchannel Flows
-
other
Microfluidic transistors for analog microflows amplification and control
Investigators
issam lakkis, principal investigator
Mohammad Cheikh, Masters, 2016
-
Mohammad Cheikh and Issam Lakkis. Microfluidic transistors for analog microflows amplification and control. Microfluidics and Nanofluidics, 20(6):1–24, 2016.
Abstract
Two microfluidic transistors for analog flow con- trol and amplification for lab-on-a-chip applications are presented. The transistors are based on the elastic mem- brane microchannel, where the flow in the microchannel between the substrate and the membrane is controlled by the pressure differences along the channel and across the membrane. Reduced-order models that capture the low- inertia dynamic behavior of the coupled fluid–structure interaction were developed to enable fast small-signal analysis of large circuits. The accuracy of the models is assessed by comparing to numerical simulations of the coupled fluid–structure interaction problem. Analog behavior (based on analytical modeling and numerical simulation) of the two devices is characterized in terms of dependence of the volume flow rate on the source–drain and gate–source pressure differences, analogous to the characterization of MOSFET operation. The characteristic curves are then used to extract the small-signal parameters (transconduct- ance and intrinsic output resistance), characterizing the dynamic response to small time-varying pressures at the gate and/or drain. The characterization enabled identifica- tion of the various static and dynamic operation regimes of the devices, including the transistive regime where the device operates as amplifier, and the capacitive (positive and negative) regimes. Finally, the dual-membrane transis- tor is used to showcase its use as a diode and a common- source amplifier in the design of a micropump that, in turn, is used for mixing of two species using pulsating flows.
​
Conclusion
Two microfluidic transistors that employ the elastic mem- brane microchannel channel are presented.
Reduced-order models that capture the low-inertia dynamic behavior of the coupled fluid–solid problem were developed to enable fast small-signal analysis of large cir- cuits. The models, when compared to detailed numerical simulations of the coupled fluid–structure interaction prob- lem over a wide range of operating pressures, proved to be most accurate when the inertia of the flow in the channel is small.
Characterization of the analog behavior of the two devices is expressed in terms of dependence of the volume flow rate versus the source–drain and gate–source pressure differences, analogous to the characteristic curves of MOS- FET operation. The characteristic curves are then used to extract the small-signal parameters (transconductance and intrinsic output resistance), characterizing the dynamic response to small time-varying pressures at the gate and/ or drain.
For the single-membrane device, the characterization revealed that, depending on these operating pressure differ- ences, the device operates as a capacitor when the mem- brane is deflected away from the channel and as a transis- tor when it is deflected into the channel. When the flow inertia is sufficiently high, a negative capacitive behavior is observed at high gate pressures. In the transistor regime of operation, the device is capable of providing large gains. Due to the narrowness of the saturation regime, the linear- ity is poor and distortion is high.
The dual-membrane transistor proposed is shown to improve linearity and reduce distortion by expanding the saturation zone. In addition to its operation as a transistor, the dual-membrane design is also shown to operate as a diode and a shutdown safety switch.
Finally, the dual-membrane transistor is used to drive a micropump equipped with two diodes. The micropump is then employed in a larger circuit that performs mixing of two species using pulsating flows.
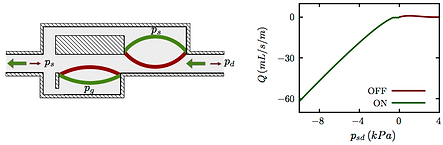
Diode configuration of the dual-membrane microfluidic transistor
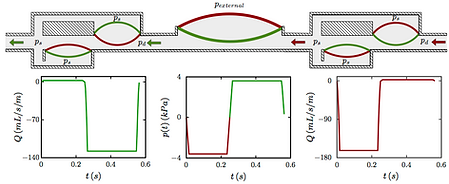
Employing the diode configuration of the dual-membrane device in the operation of a micropump
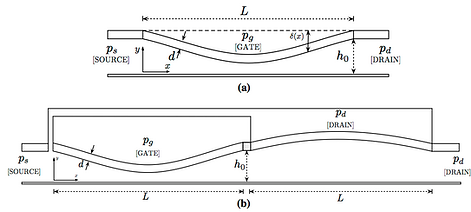
Schematics of the single- and dual-membrane microfluidic transistors. (a) Single-membrane transistor, (b) dual-membrane transistor
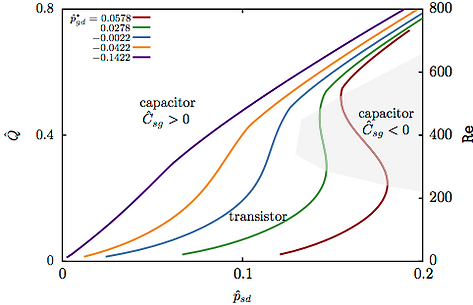
Characteristic curves of the single-membrane microfluidic transistor computed from numerical simulations of the FSI problem using Ansys
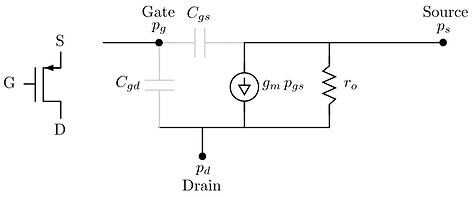
Small-signal model of the single-membrane microfluidic transistor
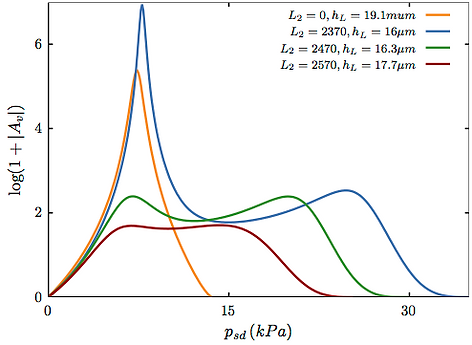
Impact of changing the length of the second membrane on the dependence of the loaded gain on the source–drain pressure dif- ference. The load is a 2D channel of length 1000 μm and height hL. L1 = 2470, pgs = 5000 Pa, pclosing = 10,00 Pa, p∗gs = −0.0581, h0 = 59 μm, d = 196 μm
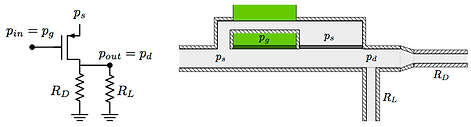
Dual-membrane device in a common-source configuration
Microfluidic mixing using pulsating flows
Investigators
issam lakkis, principal investigator
Farah Cheaib · Gacia Kekejian · Sylvie Antoun · Mohammad Cheikh
-
Farah Cheaib, Gacia Kekejian, Sylvie Antoun, Mohammad Cheikh, and Issam Lakkis. Microfluidic mixing using pulsating flows. Microfluidics and Nanofluidics, 20(5):1–19, 2016.
Abstract
Mixing of biological species in microfluidic channels is challenging since the mixing process is lim- ited by the small mass diffusion coefficient of the species and by the dominance of viscous effects, captured by the low value of Reynolds number characteristic of laminar liquid flow in microchannels. This paper investigates the use of pulsating flows to enhance mixing in microflows. The dependence of the degree of mixing on various dimen- sionless groups is investigated. These dimensionless num- bers are Strouhal number, pulse amplitude divided by base velocity, Reynolds number, location along the mixing chan- nel normalized by the channel width, channel cross sec- tion aspect ratio, and phase difference between the inlet streams. The degree of mixing, observed to experience both spatial fluctuations down the mixing channel and temporal fluctuations over a pulsation cycle at the quasi- stationary state, is shown to be most sensitive to changes in pulsation amplitude and frequency. For a fixed pulsa- tion amplitude and Reynolds number, the degree of mixing has a peak value for a certain Strouhal number above and below which the degree of mixing decreases. Increasing the pulsation amplitude improves mixing with the behav- ior becoming asymptotic at large pulsation amplitudes. The temporal fluctuations in the degree of mixing over a cycle at the quasi-stationary state decrease and the average degree of mixing increases downstream the mixing chan- nel. The fluctuations are also smaller at higher values of the Strouhal number and are generally larger for larger pulsa- tion amplitudes. This study also takes into account the rate of work input required to overcome viscous effects. While this power input is independent of the pulsation frequency, it exhibits a parabolic dependence on the pulsation ampli- tude. Finally, considering the dependence of the degree of mixing (mean and standard deviation), mixing length, and energy consumption on these dimensionless groups, examples of the trade-off that has to be made in choosing the operating conditions based on different constraints are presented.

Schematic and dimensions

Conclusion
This work presents a numerical study of mixing using pul-
sating flows in terms of dimensionless numbers that repre-
sent the pulsation amplitude (δV /V ), pulsation frequency
(St), phase angle (φ), channel height (H/W), and fluid vis-
cosity (Re). This work also takes into account the rate of
work input required to overcome viscous effect and achieve
mixing. Over the covered ranges of parameters, the pulsa-
tion amplitude (δV/V) and frequency (St) proved to have the
biggest impact on the degree of mixing down the mixing
channel. Both the average and the temporal fluctuations
in the degree of mixing over a quasi-stationary cycle are
considered. Due to the sensitivity of the solution to vari-
ous numerical parameters, care has been taken to (1) extend
the channel length to account for the effect of the exit
boundary conditions during flow reversal, (2) sufficiently
resolve space and time, (3) run the simulations long enough
to reach a quasi-stationary state. It was found that, due to
pulsation, the degree of mixing experiences spatial fluctua-
tions down the mixing channel as well as temporal fluc-
tuation over a pulsation cycle. Larger fluctuations in the
degree of mixing over a quasi-stationary cycle are observed
at lower frequencies (St) and higher pulsation amplitudes
(δV /V ). For a given W, the magnitude of these fluctuations
is smaller as H/W → 1 due to the increasing role of vis-
cous damping. We also found out that there is an optimal
pulsation frequency below and above which the mixing
deteriorates and that this optimal frequency depends on the
pulsation amplitude and channel width as f ∼ δV/4W . For low
inertia flows, the rate of work input required to overcome
friction does not depend on the pulsation frequency. As
for dependence on the pulsation amplitude, increasing the
pulsation amplitude (δV/V) yields better mixing for a given
Strouhal number. This improvement is, however, asymptotic where the large increase in energy cost may not justify the slight improvement in the degree of mixing.
Degree of mixing for the case: St = 0.2, δV/V = 10, Re = 0.2. For visual clarity, the image is magnified three times along the channel width
Reduced-Order Modeling
of Low Mach Number Unsteady Microchannel Flows
Investigators
Leila Issa, Department of Mathematics, Lebanese American University, Beirut 1102 2801, Lebanon e-mail: leila.issa@lau.edu.lb
Issam Lakkis
-
Leila Issa and Issam Lakkis. Reduced-order modeling of low mach number unsteady microchannel flows. Journal of Fluids Engineering, 136(5):051201, 2014.
Abstract
We present reduced-order models of unsteady low-Mach-number ideal gas flows in two- dimensional rectangular microchannels subject to first-order slip-boundary conditions. The pressure and density are related by a polytropic process, allowing for isothermal or isentropic flow assumptions. The Navier–Stokes equations are simplified using low- Mach-number expansions of the pressure and velocity fields. Up to first order, this approximation results in a system that is subject to no-slip condition at the solid bound- ary. The second-order system satisfies the slip-boundary conditions. The resulting equa- tions and the subsequent pressure-flow-rate relationships enable modeling the flow using analog circuit components. The accuracy of the proposed models is investigated for steady and unsteady flows in a two-dimensional channel for different values of Mach and Knudsen numbers.
​
Conclusion
​
​Reduced-order models for unsteady low-Mach-number poly- topic flow of an ideal gas in a two-dimensional parallel channel are presented for no-slip as well as first-order slip-boundary con- ditions. To first order (in Mach number), the model that satisfies the no-slip condition at solid boundaries can be represented as an analog electric circuit consisting of a set of parallel branches, each consisting of a resistance and an inductor in series. The resistors model viscous dissipation and the inductors correspond to inertia due to the time-varying term. The model can also include higher- order effects, which account for first-order slip condition at solid boundaries. The accuracy and validity of the proposed model are investigated in terms of the dynamic response of the volume flow rate for sinusoidal as well as step-function pressure history. The proposed model offers a tradeoff between speed and accuracy, which is possible by controlling the number of parallel branches included in the model.

Comparison of time evolution of mean volume-flow rate between model (- -o- -) and CFD (—–) for different values of Mach number
other
-
Leila Issa and Issam Lakkis. Reduced order models of low mach number isothermal flows in microchannels. In International ASME Conference on Nanochannels, Microchannels and Minichannels. ASME, 2013.
​​
-
Issam A Lakkis. System-level modeling of microflows in circular and rectangular channels. In ASME 2008 6th International Conference on Nanochannels, Microchannels, and Minichannels, pages 1159–1170. American Society of Mechanical Engineers, 2008.
-
Joseph Bakarji, Khoudor Keniar, Mohammad Cheikh, and Issam Lakkis. A reduced-order model of a microfluidic transistor. In ASME 2013 International Mechanical Engineering Congress and Exposition, pages V010T11A025–V010T11A025. American Society of Me- chanical Engineers, 2013.
-
Ibrahim Chamseddine, Hadi Kasab, Maya Antoun, Tawfiq Dahdah, Mohammed Mirhi, and Issam Lakkis. Analysis and design of a robust rf mems switch. In ASME ESDA conference. ASME, 2012.
-
Gunar Lorenz, Arthur S Morris III, and Issam Lakkis. Top-down design flow for moems. In Design, Test, Integration, and Packaging of MEMS/MOEMS 2001, pages 126–137. Interna- tional Society for Optics and Photonics, 2001.